Let us consider a material element
emanating from a material point located at
in the
present configuration at time
. We wish to compute
, the rate of
change of length and direction of
. Let
be the deformed position of the material point located at
in the reference configuration and
that of
. Then
Thus,
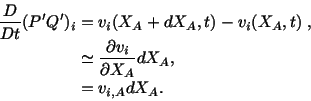 |
(3.12.1) |
Equation (3.12.1) expresses
in a material
description. To obtain
in a spatial
description, we note that since
is the velocity of a material
point
presently at the position
, therefore, if a spatial description
of velocity is employed, this velocity is given by
. Note
that
and
are, in general, different functions.
Thus,
where we have set
, the symmetric part of the velocity gradient
, is
known as the rate of deformation tensor or the strain-rate
tensor, and
, the antisymmetric
part of the velocity gradient
, is known as the spin tensor.
In the following, we give a geometric interpretation of the elements of
. Let
where
is a unit vector in the direction of
. Then
where we have substituted for
from (3.12.3). Since
, therefore
and we get
or
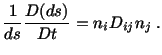 |
(3.12.6) |
If
, the right-hand side of (3.12.6)
equals
. Thus
gives the rate of change of length per unit
length known as stretching or rate of extension for a material line
presently parallel to
-axis. Similarly
and
give,
respectively, the stretching of a material line presently in the
- and
-direction. To obtain a physical interpretation of
the off-diagonal elements of
, let
and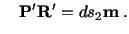 |
|
Then
|
 |
|
|
 |
(3.12.7) |
Here
is the angle between directions
and
. Thus for
and
,
the right-hand side of (3.12.7) equals
and the left-hand side equals
. Hence
equals the rate of decrease of angle
from
of two line elements presently parallel to
and
-axes, known as shearing or rate of shear. A
similar interpretation holds for
and
.
Since
is symmetric, we also have the result that there always exist
three mutually perpendicular directions (eigenvectors of
) along which
the stretching (an eigenvalue of
) is either maximum or minimum
among stretchings for
all differential elements extending from the material particle which
currently is at
.
Note that the strain-rate tensor does not, in general, equal the time rate of
change of the strain tensor. To prove this, we first conclude from eqns. (3.12.2) and (3.7.7) that
which must hold for all choices of
. Thus
or |
(3.12.8) |
where
is the spatial velocity gradient.
Differentiation of both sides of (3.8.2) with respect to time and the
definition (3.8.6)
of the strain tensor
gives
Hence
, and the strain-rate tensor
should not be confused with the time rate of change of the strain tensor
.
We now attempt to provide a physical interpretation of the spin tensor. Let
be a unit eigenvector of
, i.e.,
where
is the eigenvalue
corresponding to
. From (3.12.3), we have
where we have used (3.12.6). It states that the spin tensor
operating on a unit eigenvector of
gives the rate of change of
that unit vector. Thus the spin equals the angular velocity of the principal
axes of stretching.
The axial vector
is the vorticity vector; its direction is the axis of
spin, and its magnitude is the vorticity magnitude,
.
We now show that the spin tensor defined by (3.12.4)
does not equal the
rate of change of the rotation matrix
. Equations (3.12.9) and
(3.13.1)
give
 |
(3.13.15) |
or
where we have added and subtracted
to the
right-hand side. Equating symmetric and skew-symmetric tensors on both sides,
we obtain
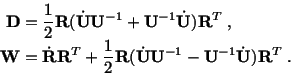 |
(3.13.17) |
These equations clearly evince that
and
.
Taking the material derivative of both sides of eqn. (3.10.2) we obtain
and, therefore,
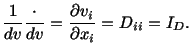 |
(3.12.13) |
In going from (3.12.11) to (3.12.12) we used
which can be obtained from eqns. (3.11.4), (3.11.5) and
(3.11.6). Thus the first principal invariant
of the rate of deformation
tensor
gives the rate of change of volume per unit volume.
We now derive an expression for the rate of change of an area element.
Rewriting eqn. (3.10.1) as
and
taking the time derivative of both sides, we obtain
In an isochoric deformation,
but
in general.
Example: Given the velocity field
find
- (a)
- the rate of deformation and the spin tensors,
- (b)
- the rate of extension per unit length of the line element
,
- (c)
- the maximum and the minimum rates of extension.
Solution
- (a)
- The matrix of the velocity gradient is
Therefore,
- (b)
- Given
. Thus
. From eqn. (3.12.6)
- (d)
- From the characteristic equation
we determine the eigenvalues of the tensor
as
. Therefore, 1 is the maximum and
the minimum rate of
extension. The eigenvector
(for
)
determined from
is
. Similarly
corresponding to
is
.
The third eigenvector is
.
Exercise: For the velocity field
find
- a)
- the rate of extension per unit length of a
material line element which in the present configuration is given by
through the point
,
- b)
- the deformation corresponding to the given velocity field if
at time
,
- c)
- the principal stretches and the deformed position of the axis
of the maximum principal stretch for the material particle which at
is at
,
- d)
- principal stretchings and their axes for the material particle
which currently is at
.